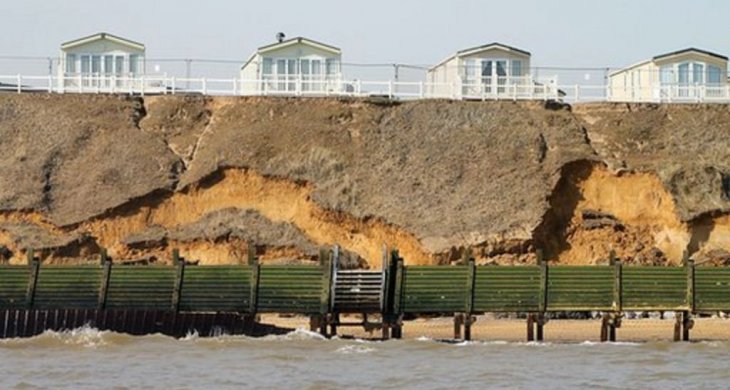
MICP for repair and protection of coastal assets

Primary Investigator: Dr Gráinne El Mountassir, University of Strathclyde
Co-Investigators: Dr Riccardo Briganti – University of Nottingham, Dr Megan Barnett – University of Nottingham
Context
With climate change predicted to contribute to rising sea levels and ultimately increased coastal flooding and erosion, it is imperative that in the UK we seek to develop innovative new approaches for protecting and managing our coastal assets, to ensure that coastal services can be maintained for communities in a changing climate. Research has shown that most beaches in Norfolk are becoming steeper and shorter1, this has a knock-on effect that beach access routes have become impassable in many locations with access ramps ending mid-air. When these assets become unusable it significantly limits the range of services the coast provides, leading to a disconnect between communities and the coastline. Conventional engineering materials (e.g. concrete) can exhibit accelerated degradation in coastal environments, result in degraded materials remaining on-site and have a high associated carbon footprint. In this project, we propose that microbially induced carbonate precipitation (MICP) could be used to repair and protect existing coastal assets making use of the abundance of sand in coastal environments to reduce transportation of materials to site, potentially reducing cost and carbon footprint of repairs. As well as repairing access ramps, MICP also has potential to be deployed to protect other coastal assets and infrastructure at risk from scouring, including sea wall defences, access steps, and retaining walls.
Microbially induced carbonate precipitation
MICP via ureolysis relies on the activity of bacteria to hydrolyse urea, producing a local pH increase and carbonate ions, promoting the precipitation of calcium carbonate (CaCO3). The precipitation of CaCO3 within porous media results in grains becoming cemented together and as such, the process has been widely investigated for soil stabilisation, with demonstrated improvements in compressive strength, shear strength, and stiffness in sands and gravels2,3. Treatment of sands via MICP has resulted in UCS values up to 11MPa3. MICP has a number of advantages over traditional grouting materials (e.g. cementitious grouts) including: (i) small particle size governed by the size of bacteria cells (~0.5m in diameter) and (ii) low viscosity of treatment fluids (≤1.1mPa.s). These properties enable excellent penetration of MICP treatment fluids into granular media and under much lower injection pressures compared to cementitious grouts.
Very few studies to date have investigated the influence of wave action on the erosion behaviour of MICP-treated soils4,5,6. These studies involve small-scale set-ups, which inevitably introduce scale effects and/or have only investigated low-energy waves4,5,6. The performance of MICP-treated sands under high-energy wave conditions remains unexplored. In this project, MICP-treated sands will be subjected to high-velocities, and in turn shear stresses, simulating coastal storm conditions, thus avoiding scale effects.
Most MICP studies to date have used the organism Sporosarcina pasteurii (S. pasteurii) to induce carbonate precipitation due to its high urease activity. Yet, microorganisms capable of inducing carbonate precipitation are ubiquitous in natural environments7. Stimulating native microbial community could not only reduce cost, remove issues associated with large-scale growth/transport of bacterial suspensions and requirement for on-site technical expertise but could also improve public acceptance of MICP as a technique, particularly in environmentally sensitive environments.
Aims & Objectives
The overarching aim of this project is to assess the potential for MICP to be deployed in coastal environments. To address this there are three main objectives:
- Quantify the impact of high-energy wave action on the erosion behaviour of sands treated via MICP using S. pasteurii (bioaugmentation).
- Explore the potential for indigenous microbial communities to induce carbonate precipitation via the ureolysis pathway (biostimulation).
- Via engagement with project partners, identify coastal sites for potential deployment of MICP technology in a follow-on full-scale demonstration project.
Work Programme
WP1: MICP treatment of porous media via bioaugmentation
Two sands (uniform sand and medium-graded very gravelly sand) with varying grain size distribution, particle shape and angularity will be treated via MICP using S. pasteurii. Previous studies have demonstrated that grain size distribution, particle shape and angularity influence MICP efficiency (in terms of strength gain), as these characteristics influence the location of calcite nucleation3. These
soil characteristics are thus also likely to influence erosion behaviour. Ultrasonic testing will be conducted on specimens to assess level and spatial distribution of MICP treatment achieved.
WP2: Physical modelling of wave action on MICP treated materials
Specimens from WP1 will be tested in a hydraulic flume (15m x 0.2m), specially adapted to test performance under dam-break flows. This approach enables the generation of energetic wave impacts, with flow velocity (and thus shear stresses) of the same magnitude as those experienced during storm conditions (i.e. several metres per s). To generate the dam-break flows, a gated water reservoir will be constructed at one end of the flume with specimens emplaced at the opposite end. The dam-break will be initiated by the rapid opening of the gate. A second gate, downstream will block the reflected wave so that specimens will be subject to isolated events. A series of these dam-break events will be generated in each experiment. These experiments will be designed to simulate wave action on an access ramp. The portion of the slope from the toe to the emerged part in the vicinity of the still water level (where maximum shear stress occurs during a bore-driven swash8), will be removable and interchangeable. This will limit the volume of sand to be treated via MICP, thus enabling repeat experiments to be conducted. The upper portion of the slope will be untreated in all experiments. Moulds will be constructed to assist emplacement of treated specimens into the flume and to ensure these remain fixed in place during experiments. Numerical simulations will be carried out during detailed design stage to optimise dimensions of the MICP treated wedge. A minimum of 3 replicates will be carried out for each material condition (Uniform sand: Untreated and Treated; Medium-graded sand: Untreated and Treated). LiDAR scanning (with mm resolution) will be conducted to evaluate specimen erosion and changes to slope profile after repeated wave events. Resistive wave gauges and high-speed cameras will also be used to investigate the evolution of the flow and the exchange of sediments between the treated and untreated portions of the slope.
WP3: Exploration of ureolytic potential of indigenous microbial species
WP3 will explore the potential for indigenous microbial communities to induce carbonate precipitation via ureolysis. Sand and soil will be sampled from locations along the Norfolk coast and microbial diversity assessed using 16s rRNA gene sequencing (MinION, Oxford Nanopore Technologies). Selected field samples will be exposed to growth media rich in urea, and changes in microbial community will be monitored by gene sequencing. Urease activity of in situ microbial communities will be compared to that of S. pasteurii. If adequate urease activity is detected in an indigenous microbial community, then as a follow-on this enrichment culture would be used to treat three soil types (including the sands from WP1) and compared against treatment using S. pasteurii in small-scale column tests, by evaluating unconfined compressive strength and calcium carbonate content.
WP4: Identification of sites for follow-on full-scale demonstration project
The project team has strong support from the project partners: Andrew Powell (Innovation Manger, Environment Agency), Rob Goodliffe (Coastal Transition Accelerator Programme Manager, North Norfolk District Council) and David Collins (Regional Business Excellence Manager, BAM Nuttall Ltd), see letters of support attached. The project partners will attend a minimum of 2 project meetings and will work with the team to (1) identify potential coastal locations for a follow-on full-scale field trial, (2) scope-out funding opportunities that could be accessed to support the field trial (e.g. Coastal Transition Accelerator Programme, Innovate UK) and (3) support dissemination of the technology & project results to other stakeholders (e.g. delivery of a Lunch ‘n’ Learn to Coastal Partnership East).
- References
- North Norfolk District Council (2009) Sheringham Coast Protection Scheme. Doc 7.
- Nafisi et al., (2020) Shear Strength Envelopes of Biocemented Sands with Varying Particle Size and Cementation Level, J. Geotech. Geoenviron. Eng., 2020, 146(3).
- Terzis, D., & Laloui, L. (2018). 3-D micro-architecture and mechanical response of soil cemented via microbial-induced calcite precipitation. Scientific Reports, 8(1), 1416.
- Shahin et al., (2020). Microbial-induced carbonate precipitation for coastal erosion mitigation of sandy. Slopes. Geotechnique Letters 10, 211-215. 5. Liu et al., (2021) An experimental study of mitigating coastal sand dune erosion by microbial- and enzymatic-induced carbonate precipitation. Acta Geotech. 16, 467–480 (2021). 6. Tsai, C.-P.; Ye, J.-H.; Ko, C.-H.; Lin, Y.-R. An Experimental Investigation of Microbial-Induced Carbonate Precipitation on Mitigating Beach Erosion. Sustainability 2022, 14, 2513.
- Zhu, T and Dittrich, M (2016). Carbonate Precipitation through Microbial Activities in Natural Environment, and Their Potential in Biotechnology: A Review. Front. Bioeng. Biotechnol. 4:4. 8. Briganti et al. (2011). Non linear shallow water modelling of bore-driven swash: Description of the bottom boundary layer. Coastal Engineering, 58(6), 463-477.
Track record:
MICP for repair and protection of coastal assets
University of Strathclyde (UoSt): The Centre for Ground Engineering and Energy Geosciences (GEEG)at the University of Strathclyde is a highly-innovative cross-disciplinary research group, that bridges the gaps between geotechnical engineering, geomicrobiology, geochemistry, geology and geophysics, with interests in (1) novel ground improvement/barrier technologies, (2) low carbon earth infrastructure and (3) energy geosciences. Staff in GEEG currently lead >£9M of UKRI/EPSRC funded projects.
Dr Grainne El Mountassir (Principal Investigator)is a Reader in Geotechnical Engineering and a UKRI Future Leaders Fellow. Her research interests are in the application of microbial processes within geotechnical engineering. In particular, she has been investigating microbially induced carbonate precipitation for 13 years. She is currently PI of Soil-mycelia systems for slope stabilisation(UKRI £1.3M, MR/V025376/1) and was formerly PI of Grouting of well leakage and migration pathways: biogrouting and colloidal silica (Oil & Gas Technology Centre, £235k) and Fungal Hyphal Networks for Ground Improvement(EPSRC, £99k, EP/N035526/1).Although El Mountassir has experience in leading UKRI research projects, to date she has not led any multi-institutional research projects.
University of Nottingham (UoN):The Environmental Fluid Mechanics and Geoprocesses (EFMG) research group at the University of Nottingham undertake field-, laboratory-, theoretical-and numerical-based research with expertise inflow in granular porous media, coastal sediment transport, extreme waves, ice and avalanche dynamics. The Fluids Laboratory within EFMG is equipped with a hydraulic/wave flume, with a vast array of instrumentation including resistive level gauges, pressure transducers, strain gauges, and high-speed cameras.
Dr Riccardo Briganti(Co-investigator), is an Associate Professor in Coastal Engineering. He is an expert in modelling coastal morphodynamics and sediment transport. His research focuses on understanding physical processes occurring within individual wave cycles in the nearshore region and fast evolving flows. He previously held an EPSRC Career Acceleration Fellowship(EPSRC, £563k, EP/I004505/1)and was Co-I in the EPSRC funded project Flood MEMORY(EPSRC, £1.8M, EP/K013513/1. He currently supervises 2 PhD students via the NERC-funded ENVISION DTP(NE/S007423/1)and he is Co-I in the Horizon Europe Doctoral Network SEDIMARE(EP/X027864/1).British Geological Survey(BGS):The BGS is a world-leading geological survey and global geoscience organisation, focused on public-good science for government and research to understand earth and environmental processes. BGSare the UK’s premier provider of objective and authoritative geoscientific data, information and knowledge to help society to use its natural resources responsibly, manage environmental change and be resilient to environmental hazards.
Dr Megan Barnett(Co-investigator)is a geomicrobiologist with expertise in microbial detection in extreme and low biomass environments, molecular biology and characterisation of microbial communities. She is currently working on Bioprecipitation for Geosciences, one of 23 projects funded under the UKRI International Geoscience Research & Development programme. She recently completed work on the Horizon 2020 project: Re-using EXisting Wells forCO2storage operations(ERA-ACT REX-CO2), where she investigated microbial remediation of former oil and gas wells.
Relevant recent publications: Mintoet al. incl. El Mountassir (2019). Development of a reactive transport model for field-scale simulation of microbially induced carbonate precipitation, Water Resour. Res. 55(8): 7229-7245; Tobler et al. incl. El Mountassir(2018). Microscale analysis of fractured rock sealed with microbially induced CaCO3 precipitation: influence on hydraulic and mechanical performance, Water Resour.Res., 54(10): 8295-8308;El Mountassir et al. (2018). Applications of microbial processes in geotechnical engineering, Chp.2, Adv.Appl.Microbiol.104:39-91; Briganti et al. (2022).Wave overtopping at near-vertical seawalls: Influence of foreshore evolution during storms, Ocean Eng.,261: 112024; Williams et al. incl. Briganti(2019).Experimental analysis of wave overtopping: A new small scale laboratory dataset for the assessment of uncertainty for smooth sloped and vertical coastal structures, J. Mar.Sci. Eng.,7(7),217;O’Donoghue et al. incl. Briganti(2016). Intra-swash hydrodynamics and sediment flux for dam break swash on coarse-grained beaches,Coast.Eng.,112:113-130;Barnettet al (2023). Groundwater microbiology of an urban open-loop ground source heat pump with high methane, Groundwater,https://doi.org/10.1111/gwat.13291;Barnett et al. (2021). Screening microorganisms for remediation of wells via carbonate precipitation. SINTEF Proceedings7, p362-365.